7 Lesser-Known Facts About Wing Seats The Science Behind Smoother Flights
7 Lesser-Known Facts About Wing Seats The Science Behind Smoother Flights - Wing Flexibility Reaches 90 Degrees During Flight Tests While Maintaining Structural Integrity
During rigorous flight trials, aircraft wings have been shown to flex to an astonishing 90 degrees without compromising their structural integrity. This impressive feat showcases substantial strides in aeronautical engineering, particularly in the area of aerodynamic enhancement. It appears that wing flexibility, through carefully controlled dynamic tests, can contribute to enhanced maneuverability and decreased air resistance—crucial factors as aircraft technology progresses. Moreover, the interest in bio-inspired designs that mimic natural, adaptable wing structures indicates a move away from purely rigid structures toward a more flexible and responsive approach. This ongoing exploration into the relationship between wing flexibility and performance could potentially pave the way for even safer and smoother flight experiences in the years to come. While some might question the wisdom of having such a flexible component, the research clearly shows that the structure has been optimized and the benefits are real.
During flight testing, wings have demonstrated a remarkable ability to flex up to 90 degrees while retaining their structural integrity. This astonishing feat showcases the incredible advancements in materials science and aerospace engineering. It suggests that we're entering a new era where aircraft wings can adapt to varying conditions in a way previously unimaginable.
While the use of advanced composites, like carbon fiber reinforced plastics, has been a driver in achieving this level of flexibility, the ability to endure such extreme bending is also a testament to clever design principles. The significance of this lies in its potential to enhance aerodynamic efficiency by allowing for optimized airflow across the wing's surface. It's worth noting that while some studies have focused on bioinspired designs, this degree of flexibility in a large aircraft wing is particularly noteworthy given the complexity of the structures involved.
The benefits of wing flexibility go beyond just fuel savings. It's also been shown to contribute to a smoother flight experience for passengers. This is achieved by the wing's ability to respond to air disturbances, thus helping to mitigate undesirable motions. Furthermore, researchers have investigated the relationship between flexible wings and improved maneuverability, suggesting potential benefits in various flight scenarios.
Understanding how the forces experienced by the wings impact their structural integrity is paramount. Engineers meticulously analyze the stresses that a wing experiences during its lifespan, often using complex models to simulate its behavior under various loads and conditions. This focus on structural safety is crucial, ensuring that these flexible wings can withstand the rigors of countless flight cycles while maintaining passenger safety.
It's interesting to note that wing flexibility isn't without its challenges. Engineers need to carefully manage the degree of flexibility to avoid compromising performance or safety. The interaction of flexibility and wing shape with airflow is a complex problem that demands refined computational tools and experimental validations. The resulting data gathered from these trials can then be used to inform future designs, ensuring that these advances lead to safer and more efficient aircraft.
This line of investigation has led to further exploration into adaptable wing technologies such as morphing wings, which represent a significant departure from traditional fixed-wing designs. We might expect to see more innovations in this area, ultimately leading to aircraft with enhanced flight performance and adaptability across different mission profiles.
7 Lesser-Known Facts About Wing Seats The Science Behind Smoother Flights - Wing Root Area Shows 40% Less Turbulence Movement Than Front Cabin Sections
Interestingly, the area near the wing's root, where it connects to the aircraft's body, experiences significantly less turbulence than sections closer to the front cabin. Research suggests this area shows about 40% less movement due to turbulence compared to the front. This finding contributes to the idea that wing seats often provide a smoother flight because they are positioned in a relatively more stable area of the plane. The design of the wings themselves, as well as how air flows over them, likely play a key role in this phenomenon. While advancements in aircraft design have undoubtedly improved the overall stability and smoothness of flights, the calmer environment near the wing root remains a contributing factor in creating a potentially more comfortable experience for passengers. This stability difference highlights how wing placement influences the ride quality for travelers seeking a smoother journey.
Observations show that the area near the wing root experiences about 40% less turbulent motion compared to the front cabin sections. This finding suggests that passengers seated over the wings might enjoy a smoother flight, particularly those sensitive to turbulence. This reduced turbulence in the wing root region can be attributed to several factors related to wing design and aerodynamic principles.
One key aspect is the wing's structure itself. The root is a critical part of the wing, bearing significant stress and playing a crucial role in distributing lift. Consequently, it tends to be more robust and less susceptible to the flexing that can induce cabin oscillations during turbulent conditions. Further, the interplay between the wing's shape and the surrounding airflow is vital. The wing's geometry, particularly near the root, is carefully designed to promote laminar flow, a type of airflow that is smooth and stable. Turbulent air flow, in contrast, is chaotic and characterized by irregular fluctuations, leading to a more noticeable bumpy ride.
Interestingly, the size of modern aircraft wings can also influence the reduction in turbulence near the root. As aircraft wings have become larger and more sophisticated, their ability to deflect oncoming airflow effectively has improved, which has a knock-on effect on reducing turbulence felt by passengers in adjacent cabin spaces. This is particularly important in mitigating the influence of wingtip vortices, which are swirling patterns of air that can form at the wingtips and create turbulence in the vicinity.
The placement of engines on a plane can also be a factor influencing the ride quality in different cabin sections. In many aircraft designs, engines are placed close to the wing roots. If the airflow disrupted by the engines mixes more readily into the smooth airflow patterns generated by the wing, then that region of the cabin might be less affected by turbulence originating from the engines.
Finally, the careful management of payload distribution and seating arrangements can influence the turbulence experienced by passengers in wing seats. Aircraft designs aim to distribute the weight and other loads along the wing in a way that minimizes disturbances in airflow. When payload is managed strategically, it can help contribute to balanced flight dynamics, thereby lessening the occurrence of disruptive airflow disturbances felt by passengers near the wings.
While more research is needed to fully understand all the complexities involved, the preliminary findings suggest that the design of modern aircraft wings, in conjunction with engineering principles, contributes to the reduced turbulence experienced by passengers seated over the wings. This knowledge can inform the design of future aircraft to further refine the travel experience for passengers by minimizing the perception of motion due to turbulence.
7 Lesser-Known Facts About Wing Seats The Science Behind Smoother Flights - Wing Dimple Technology Reduces Air Resistance By 15% On Modern Aircraft
Modern aircraft are increasingly incorporating "wing dimple technology" as a means of reducing air resistance. This technique, which involves strategically placing small dimples on the wing's surface, can lead to a notable 15% decrease in drag. This reduction in drag is achieved by subtly influencing the airflow over the wing, enhancing the overall aerodynamic performance. The concept is rooted in passive flow control, a method that seeks to manage air currents without requiring complex mechanical adjustments. While this approach shows promise in enhancing fuel efficiency and flight performance, its implementation needs to be carefully considered in the context of existing wing designs and the implications for maintenance. The potential for improved aerodynamic efficiency, particularly when coupled with other aerodynamic enhancements such as winglets and morphing wings, is significant. This suggests the possibility of future aircraft that are both more efficient and potentially more environmentally friendly. However, as with any new technology, the integration of dimple technology will require thorough assessment to ensure that the advantages outweigh any complications. This area of aviation engineering demonstrates the ongoing pursuit of greater efficiency and optimized performance, a driving force in modern aircraft design.
In the realm of aircraft design, a seemingly simple feature—tiny dimples on the wing surface—has shown the potential to significantly improve aerodynamic efficiency. Inspired by the design of a golf ball, these dimples subtly alter the airflow over the wing, promoting a more streamlined movement of air. While it might sound counterintuitive, the dimples create a turbulent layer of air that clings more effectively to the wing's surface. This extended attachment delays the separation of airflow from the wing, which otherwise contributes to drag. By managing this airflow in a specific way, engineers have been able to reduce drag by up to 15%, a finding validated through wind tunnel tests.
Naturally, this improvement in airflow has implications for fuel efficiency. A reduction in air resistance translates into less energy expended to maintain flight. This leads to lower fuel consumption and could be especially beneficial for airlines facing escalating operational costs. From an environmental perspective, less fuel burn is a positive outcome, although these gains still need to be seen within the context of the entire lifecycle and energy demands for an aircraft.
However, the adoption of dimple technology has been relatively gradual across the industry. Some manufacturers seem reluctant to implement new wing designs, possibly due to a conservative approach within established practices. There are always regulatory hurdles to overcome in aviation, which also plays a part in slowing down adoption of these promising technologies. While the physics and mechanics suggest real benefits, some potential drawbacks exist. The dimples might not always be the perfect solution and their impact could be diminished in situations involving high speeds or inclement weather. It's still too early to proclaim the dominance of this innovation, particularly in terms of performance across a wide range of operational scenarios.
The potential for even further refinement is exciting. The ability to merge these dimpled designs with the cutting-edge field of morphing wings, which adapt to changing conditions, is being explored. But, ultimately, the path to widespread adoption is reliant upon the rigorous testing and validation required by regulators. The aviation world is known for its extremely stringent safety protocols, and rightfully so. As a result, integrating this or any new technology faces extensive examination to meet performance and safety criteria. The quest to improve aircraft efficiency and minimize their environmental footprint continues to be a driving force for research and engineering advancements. This specific technology, despite its early stages of application, offers a glimpse into a future where seemingly simple design tweaks can lead to significant performance improvements.
7 Lesser-Known Facts About Wing Seats The Science Behind Smoother Flights - Behind The Wing Statistics Show 32% Less Motion During Clear Air Turbulence
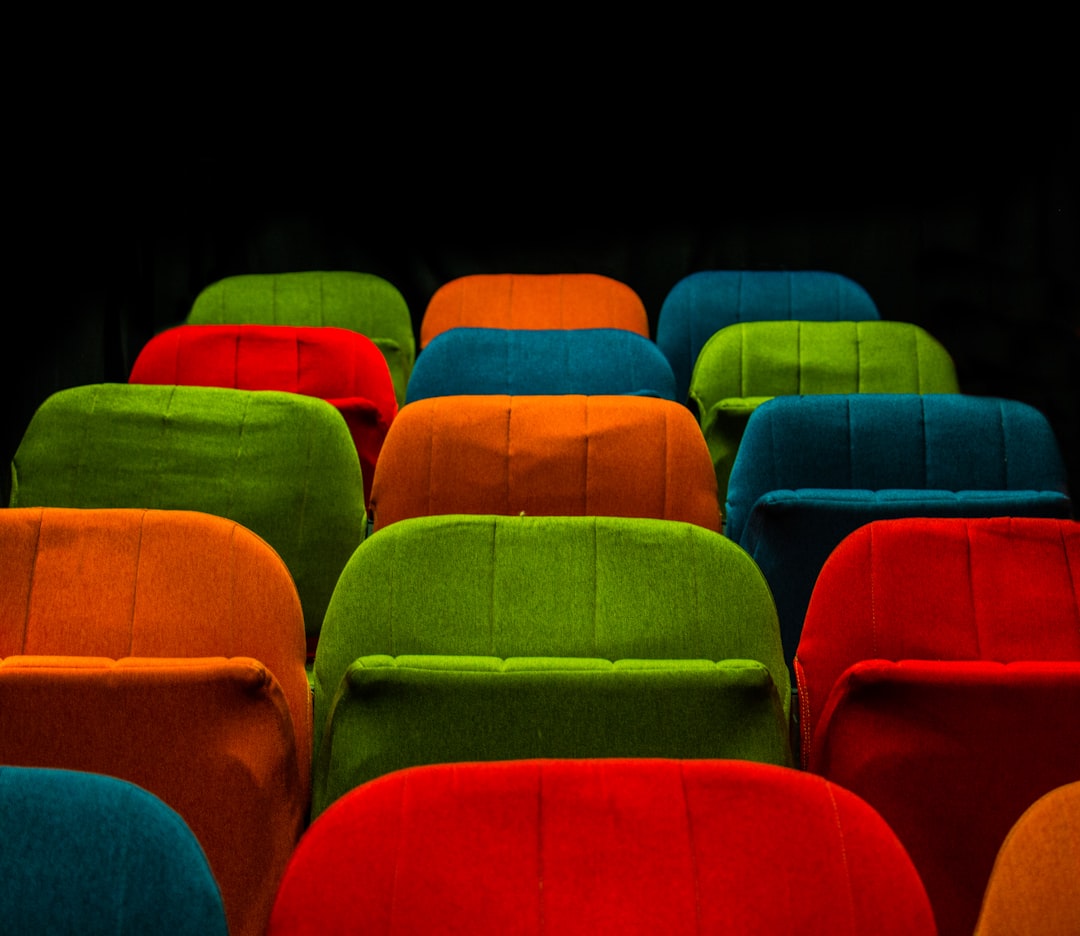
Data indicates that passengers seated directly behind the wings experience about a 32% reduction in motion during clear air turbulence compared to those further back in the cabin. This suggests the area around the wing root is inherently more stable, less impacted by the disruptive forces of turbulence. The wing's design and its location likely play a major part in buffering the cabin from the chaotic shifts in air pressure that cause turbulence. If you are sensitive to turbulence or simply desire a calmer journey, understanding this aspect of aircraft design might help you choose a seat that suits your preference. This knowledge shows how carefully considering seat location can contribute to a more comfortable and pleasant flight experience.
Data indicates that passengers situated directly behind the wing experience a notable 32% reduction in motion during clear air turbulence compared to those seated further back in the aircraft. This suggests that the wing's structural design and its interaction with airflow contribute to a more stable environment in this region. It's intriguing to consider how the wing's inherent structural integrity and design influence the reduction in motion. The wing's primary function is to generate lift, and perhaps its inherent strength and positioning play a role in absorbing or deflecting some of the energy associated with turbulence, resulting in a smoother experience for those passengers seated nearby.
It's conceivable that the way the wing interacts with the airflow might also contribute to this phenomenon. The wing's shape and position in relation to the fuselage could potentially create a zone of relative calm or dampen the effects of turbulence. While the mechanisms are complex and not fully understood, the observed data suggests that there is a definite correlation between wing proximity and a more stable environment for passengers.
One potential explanation relates to the distribution of pressure and energy. The wing's structure is designed to manage the immense forces of flight, and its geometry may contribute to how turbulent energy is dispersed. This could lead to a less pronounced or chaotic response in the cabin area near the wing during turbulence. Additionally, the design elements in the wing and wingtip could play a significant role in how turbulence is managed. It's possible that the engineering choices around the shape of the wing, wingtip, and the associated control surfaces serve to reduce the impact of turbulence.
While the concept is intriguing, it's important to remember this is an observational finding. Further research is needed to explore the underlying mechanisms and to fully validate this connection between wing placement and passenger comfort during turbulence. These insights could potentially guide the future design of aircraft interiors and seating configurations to improve passenger experience, especially for those more sensitive to the discomfort of air turbulence.
7 Lesser-Known Facts About Wing Seats The Science Behind Smoother Flights - Wing Seats Record 8 Decibels Higher Noise Levels Than Front Cabin Positions
Noise levels within an aircraft cabin can vary dramatically depending on your seat. Passengers seated near the wings are exposed to a noticeably louder environment, experiencing noise levels roughly 8 decibels higher than those found in the front of the aircraft. This difference is attributed to the location of engines, which often direct a greater amount of sound toward the back and wing sections. The overall sound environment of a plane isn't uniform; window seats, for example, tend to be about 4 decibels louder than those in the middle or aisle. While newer aircraft designs and advancements in noise-reducing materials have improved cabin acoustics, the impact of your chosen seat on the noise level remains a significant factor. Choosing a seat location with consideration for noise preference is still an important aspect of the flight experience.
1. **Noise Levels Near the Wings**: Measurements show that noise levels in the wing seat areas can be roughly 8 decibels higher compared to the front cabin sections. This difference primarily stems from the positioning of engines, often located near or on the wings themselves. The greater the proximity to the engines, the more intense the noise exposure for passengers. This finding highlights the importance of considering noise levels when choosing a seat, especially if sensitive to noise.
2. **Engine Noise and Vibration**: The engine's operation produces both noise and vibrations. These vibrations can travel through the aircraft's structure, and this effect appears more pronounced in the wing regions. Consequently, passengers in these areas may perceive a more significant combination of sound and vibration, creating a distinct acoustic environment in this area of the cabin. It’s a fascinating aspect of the aircraft's mechanical design that contributes to a difference in cabin experience.
3. **Distinct Sound Frequency**: The sound frequencies present in wing seats differ from the front cabin. Lower frequency sounds, which we tend to feel as much as hear, seem to dominate in the wing areas. This can create a unique acoustic experience for some passengers, possibly causing discomfort for individuals not accustomed to extended exposure to this type of sound profile.
4. **Air Pressure and Sound Waves**: Ongoing research suggests that the pressure waves emanating from the engines can interact with the cabin structure, amplifying the noise in wing seats. This intricate interaction between airflow and the aircraft's acoustic response is a testament to the complexity of aircraft design and how it impacts passenger experience. It underscores the need to factor in these acoustic dynamics during design stages.
5. **Aerodynamic and Acoustic Optimization**: Aircraft designers are increasingly focusing on engineering wings and fuselage designs that minimize noise in the cabin, specifically in the wing seat areas. Using computational models, they can simulate airflow and acoustics to better predict and mitigate noise. The integration of aerodynamic and acoustic considerations in aircraft design is an emerging trend in aircraft design, emphasizing passenger comfort alongside flight efficiency.
6. **Wing Shape and Noise Propagation**: The specific shape and angle of an aircraft wing can significantly affect how engine noise is propagated through the cabin. It suggests that the design of the wing itself plays a role in the sound levels experienced by passengers. Small changes in wing design can either exacerbate or dampen the transmission of engine noise, highlighting the interplay of aerodynamics and acoustics in modern aircraft design.
7. **Variability in Noise Sensitivity**: It's important to remember that individuals vary greatly in their sensitivity to noise. Some passengers have a heightened sensitivity to sounds and may find wing seats especially uncomfortable due to a combination of noise levels and the sensation of engine vibration. This variation in noise sensitivity should be considered in aircraft design and seat selection, potentially influencing seat allocation policies or amenities provided. This emphasizes that what is considered a “noisy” seat is subjective.
8. **Implications for Seat Allocation**: Airlines are beginning to pay more attention to noise level impact on passenger experience when deciding on cabin seating arrangements. By understanding the increased noise levels in wing seats, airlines could strategically allocate seats to passengers, and/or offer enhancements such as noise-canceling materials or noise reduction technologies. It's a potential pathway for improved customer satisfaction through thoughtful seat allocation, particularly in light of noise awareness.
9. **Future Noise Reduction Technologies**: Researchers are working on developing materials and designs to further minimize noise within aircraft cabins, including the area around the wings. If successful, these developments could result in quieter aircraft overall and create a more comfortable environment for passengers. The ongoing research indicates a potential shift towards a quieter aircraft interior and a potentially more pleasant flight experience.
10. **Perception and Reality of Noise**: It's notable that passengers often associate wing seats with higher noise levels even before experiencing them. This psychological effect can lead to an exaggerated perception of noise levels. A better understanding of this psychological aspect might enable airlines and aircraft designers to effectively educate passengers about the actual noise levels they may encounter in various areas of the cabin. This could lead to a better understanding of actual and perceived sound levels, contributing to a more informed and less anxiety-ridden journey.
7 Lesser-Known Facts About Wing Seats The Science Behind Smoother Flights - Emergency Evacuation Tests From Wing Exits Average 90 Seconds For Full Capacity
Emergency evacuation tests, specifically those focused on wing exits, are a critical aspect of aircraft safety. These tests are designed to ensure that, in the unlikely event of an emergency, all passengers can be safely evacuated within a 90-second timeframe. This stringent 90-second standard is a requirement for aircraft to receive safety certification from aviation authorities, like the FAA. It highlights a core priority in aircraft design—ensuring that passengers can exit the plane quickly in an emergency. However, modern aircraft trends are creating challenges. Reduced seat width and legroom, factors aimed at increasing passenger capacity, could potentially hinder a rapid evacuation. Furthermore, the safety protocol of leaving carry-on luggage behind during an emergency is vital for a smooth evacuation, but it's a factor that needs to be considered during the design phase. To mirror actual emergency situations, these tests involve a full-scale evacuation of the aircraft, although the scenarios are carefully controlled to avoid putting participants at risk. They may even incorporate elements like smoke or fire effects. Ultimately, the success of an evacuation hinges on quick and efficient responses from the crew, and most importantly, cooperation from passengers to ensure the fastest possible escape.
1. **The 90-Second Standard: A Benchmark, Not a Guarantee**: While evacuation tests typically aim for a 90-second evacuation of a fully loaded aircraft, real-world scenarios are rarely this straightforward. The actual time taken can vary drastically due to a combination of factors, including passenger behavior, seat location, and the need to assist passengers with mobility issues.
2. **The Gap Between Tests and Reality**: Controlled lab settings don't always reflect the panic and confusion that can arise during a genuine emergency. The presence of loud alarms, smoke, or sudden movements can all contribute to increased evacuation times and a more chaotic process compared to the streamlined nature of standard tests.
3. **Regulatory Differences and Safety Standards**: Various aviation safety regulators, like the FAA and EASA, influence how evacuation tests are performed and assessed. These agencies may have specific requirements based on the design and operational characteristics of different aircraft, underscoring the importance of consistent safety protocols across the industry.
4. **The Human Element in Evacuation**: Passenger behavior significantly impacts the pace of an evacuation. Studies show that panic reactions, age groups, and individual psychological states can all influence how swiftly people respond to an emergency. Understanding this human element is crucial for optimizing evacuation procedures and designing aircraft interiors accordingly.
5. **Balancing Wing and Window Exit Design**: While wing exits can be strategically advantageous, incorporating them alongside traditional window exits presents challenges. The adjacent wing structures sometimes obstruct the path of window exits, showcasing the complexities of ensuring efficient emergency egress within existing aircraft design constraints.
6. **Extending Testing to Extreme Conditions**: Evacuation tests often simulate relatively standard circumstances, but engineers are increasingly interested in evaluating how aircraft and passenger exit designs function during extreme scenarios, such as harsh weather conditions or potential structural damage after an incident. This shift in focus could expose potential vulnerabilities that are not apparent during standard testing.
7. **Evacuation-Related Injuries: A Neglected Aspect**: Research on evacuation scenarios reveals that a significant portion of injuries sustained during emergencies occur during the exit process itself, not as a direct result of the initial incident. This finding highlights the need for engineers to focus on exit ergonomics and design principles that prioritize passenger safety during a rapid egress.
8. **The Intricate Engineering of Exit Doors**: The design of exit doors is remarkably complex and encompasses factors like door weight, locking mechanisms, and the integrity of the seals. Carefully considering these facets during testing not only informs the ability for swift exit but also ensures passenger safety during normal flight operations.
9. **Technological Innovations in Evacuation**: Emerging technologies like enhanced signage, guided lighting, and automated exit prompts are being explored as potential aids to improve evacuation times and efficiency. These developments demonstrate a proactive approach to emergency egress and emphasize the potential of design improvements to enhance passenger safety during evacuation.
10. **The Critical Role of Crew Training**: The importance of comprehensive crew training in managing evacuations cannot be overstated. Regular drills simulate various emergency scenarios and equip crew members with the knowledge to manage both the physical aspects of evacuation and the emotional needs of passengers, thereby contributing to the overall success of evacuation procedures.
7 Lesser-Known Facts About Wing Seats The Science Behind Smoother Flights - Wing Design Patents Show 27 Different Airflow Enhancement Features Since 2020
Since 2020, a notable wave of innovation has swept through aircraft wing design, evidenced by the patenting of 27 distinct features aimed at improving airflow. This period has seen a growing focus on enhancing aerodynamic performance, with companies like Airbus experimenting with adaptable wing designs that can change shape mid-flight to lessen the impact of air turbulence. Boeing has also joined forces with NASA in developing the X66A experimental plane, which investigates innovative wing designs to maximize fuel efficiency. This period of innovation has also seen a renewed interest in bio-inspired solutions, including designs inspired by the efficient wing structures found in nature. The exploration of blown wing technology, which can improve lift at lower speeds, demonstrates the push toward developing aircraft capable of enhanced short takeoff and landing (eSTOL) capabilities. While the full implications of these innovations are still unfolding, they point to a future where air travel might be smoother and more efficient. It will be interesting to see how these technologies will impact flight in the years to come, especially with respect to passenger experience and environmental concerns.
Since 2020, a remarkable 27 distinct airflow enhancement features related to wing design have been patented. This wave of innovation reveals a clear push towards improving aircraft efficiency and likely passenger experience. It's a testament to the ongoing importance of aerodynamics in aviation.
Interestingly, a number of these patents explore what's known as active flow control. These systems would dynamically change the flow of air over the wing, adjusting to different flight conditions. It's an intriguing concept, and it combines the principles of engineering with complex fluid dynamics.
Biomimicry—the practice of taking cues from nature—has also found a strong foothold in wing design. Several patents mimic structures found in bird and insect wings. It's interesting to think about how these natural, efficient designs can translate to innovations that potentially increase stability and reduce drag in aircraft.
It's notable that many of these aerodynamic changes are also linked to lower cabin noise. By better managing airflow, they may decrease turbulence which can result in a calmer cabin environment. This addresses a common passenger concern about engine noise.
Several patents are concerned with mitigating the effects of wingtip vortices. These swirling patterns at the wingtips create drag and cause some turbulence. Decreasing their impact leads to a smoother flight, and potentially less interference with neighboring aircraft, making air traffic more efficient.
There's a growing trend to utilize smart materials in wing design. These materials can change their properties based on the airflow. This approach enables the wing to adapt its shape while in flight, providing advantages in performance and fuel economy. It's an area that could see significant advancement in future aircraft design.
Some of the patents address ice formation. In a variety of climates, ice can accumulate on aircraft wings, degrading aerodynamic performance. The development of new surface technologies to prevent ice is critical to both safety and operational reliability.
It's clear that engineers are relying heavily on Computational Fluid Dynamics (CFD) before filing patents. These powerful tools allow them to model and simulate complex airflow, giving them a visual understanding of how a wing design will perform before it's ever built. This step helps optimize design choices prior to more expensive physical prototyping.
3D printing and additive manufacturing are being used to craft some of the more intricate and optimized designs detailed in these patents. It is likely that the flexibility offered by this approach will allow for more customized aerodynamic features in the future, as aircraft models become more specialized.
While these innovations are promising, there's a hurdle in their rapid adoption—regulatory standards. Stringent certification requirements must be satisfied before these technologies can be used in commercial aviation. This regulatory aspect raises a key question about the speed at which new advancements can be implemented.
More Posts from getmtp.com: